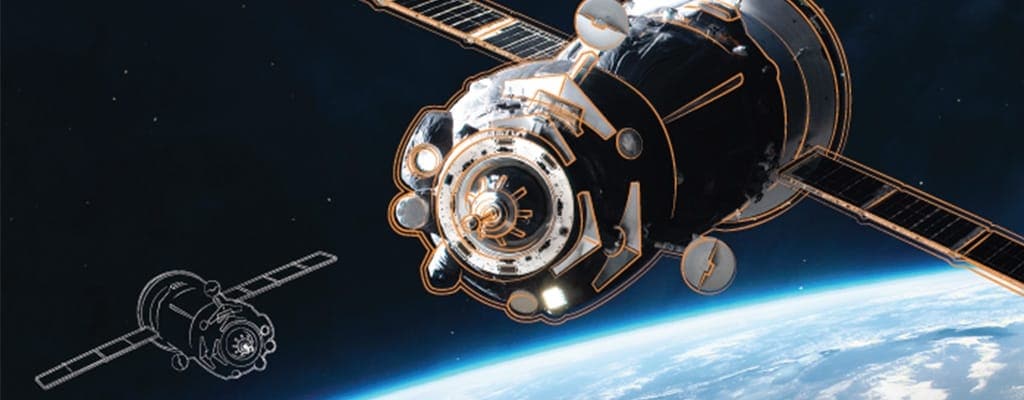
Trend Insight
Factors That Affect Spacecraft Connectors
Understanding the factors that influence the cost and performance of spacecraft connectors can help meet mission-critical objectives for LEO satellites.
What Are the Requirements for Space-Grade Connectors?
Electronic and electrical components and their interconnects undergo extreme stress during the launch and orbit insertion of satellites into low Earth orbit (LEO). Addressing these challenges is essential for the many private space companies and government-owned space agencies that are pioneering the LEO-satellite frontier.
On LEO missions, interconnects must meet demanding requirements for factors like vibration, electrostatic discharge, outgassing, extreme temperatures (from -270°C to 200°C) and size-weight-and-power (SWaP), among others. Understanding the factors that influence the cost and performance of spacecraft connectors can help designers meet mission-critical objectives for launch vehicles, LEO satellites and constellations employed in today’s space industry.
Minimizing Size and Weight
In recent years, the cost of launching a kilogram of payload into space has dropped significantly, due in part to the reusability of major launch vehicle components.
However, reducing interconnect size and weight involves trade-offs. As dimensions become smaller, it becomes more difficult to maintain accurate tolerances. And as materials become less massive, it is difficult to maintain strength, mating retention forces and resistance to mechanical stress.
Material properties, quality and tolerances must be validated to assure the high performance of lightweight, miniaturized spacecraft connectors. Everything in the supply chain — including raw materials and assembled parts — must meet defined standards developed by organizations, including:
- NASA
- U.S. Department of Defense (DoD)
- SAE International
- International Organization for Standardization
- European Space Agency
- Indian Space Research Organization
- Japan Aerospace Exploration Agency
After final assembly, parts must be individually tested for zero defects to ensure continuous operation in a harsh space environment.
Withstanding Vibration, Acoustic Loads and Shock
While there is minimal vibration in space, vibration is significant during launch. Side to side (lateral axis) and forward to backward (thrust axis) motion can lead to misalignment or breakage in the contact area.
Damage typically occurs at loose or improperly crimped connections, wire defects and fatigue points, or where insufficient clearances or poorly routed points allow scrape abrasion.
To endure the effects of extreme vibration, acoustics and shock, many factors must be accounted for, such as:
- Latching forces
- Locking mechanisms
- Wire fatigue
- Weld/solder joints
- Potting materials
- Insulation
Withstanding Extreme Temperatures
The temperature in the vacuum of space is 2.725 Kelvin (-454.72°F/-270.4°C). However, onboard systems in LEO satellites operate within a -65°C to 125°C range depending on orbit height, solar heat gain moderated by satellite spin and heat generated by electronics. Propulsion system components, external latches, sensors and solar panels are subject to more extreme temperatures.
Exposure to thermal cycling and to high peak and low cryogenic temperatures produces stresses in metal, glass and polymer materials that aggravate minor imperfections, compromise strength and degrade performance. Higher temperatures also increase vapor pressure and the rate of chemical reaction, which increase outgassing.
Materials must be selected for every component — from wire and cable to connectors and relays — that can accommodate peak temperatures and thermal cycling stress.
Minimizing Outgassing
Outgassing occurs when gases trapped in nonmetallic materials — such as polymers used in connector inserts, seals, adhesives or potting materials — are released in the vacuum of space or by high temperatures. The released gases can condense on and contaminate sensitive surfaces, eventually degrading the performance of charge-coupled device (CCD) sensors in satellites, thermal radiators or solar cells. Moreover, the gas discharged from materials contributes to regimes of critical pressure during ground test and flight that create the environment for a corona (partial) discharge or a Paschen (full) electrical breakdown event.
NASA ASTM E595-77/84/90 testing and the MIL-W-22759 (M22759) | SAE AS22759 specification cover a material’s performance when exposed to high heat or a vacuum. Anything considered a low-outgassing material must meet requirements for a total mass loss of 1.00% or less and a collected volatile condensable material (CVCM) of 0.10% or less.
Inorganic materials are immune from outgassing and can be used, for example, in connector shells employing aluminum with an electroless nickel finish. Materials can also be selected that meet NASA requirements for low outgassing in thermal oven bakeout testing.
Controlling Electrostatic Discharge (ESD)
Reports indicate that 54% of spacecraft anomalies or failures are caused by electrostatic discharging and charging.
On Earth, a material can become electrically charged when electrically dissimilar materials rub together. But in wires and cables used in spacecraft, a static charge can be created by the impact of charged particles on the material. Beyond low-Earth-orbit, ESD concern becomes greater for satellites in geosynchronous Earth orbit (GEO) because of higher charge density in deep space. When the charge builds up in wire and cable of electrical interconnection systems, a sudden discharge can damage connected logic circuits, electronic instruments and computer chips.
The speed and size of an electrostatic discharge are determined by a material’s ability to hold a charge (capacitance) and its ability to reduce the flow of electrons (resistance). One solution is to employ metallic shielding to create a path that promotes electron flow to dissipate the charge.
Reducing Electromagnetic Permeability
Magnetic permeability — signified by the Greek letter Q — denotes a material’s ability to form a magnetic field. Materials with high Q become highly magnetic when a magnetic field is applied; low Q materials are less responsive and exhibit lower levels of magnetism. While materials with high Q are suitable for some space applications, strongly magnetic materials in electronics can degrade circuit performance, signal clarity and instrumentation accuracy.
Space-grade connectors and other interconnect components with a maximum permeability of 2 Q or lower are generally required. To ensure low Q, designers must avoid ferromagnetic materials, such as carbon steel commonly used in older MIL-DTL-24308 D-Subminiature connectors.
Non-ferromagnetic materials — such as aluminum, copper, titanium and austenitic stainless steel — generally exhibit low Q. For example, aluminum with an electroless nickel finish is often used as a connector shell material.
Space applications can also require interconnects that exhibit low residual magnetism, also known as low magnetic field retention. For example, brass shell materials with gold-over-copper flash finish are typically used in D-Subminiature connectors.
Controlling Corrosion
Corrosion affects interconnects differently in space than on Earth. During spacecraft manufacturing and storage on Earth, galvanic corrosion can occur when two dissimilar plating materials are in direct electrical contact with each other in the presence of an electrolyte. In the contact area, the less resistant material acts as an anode, and the other material acts as a cathode creating a galvanic cell that attacks — or corrodes — the anode.
In space at LEO altitudes between 200 km and 700 km, UV excitation of the remaining O2 molecules at the fringe of the atmosphere forms monatomic oxygen. Atomic oxygen (ATOX) corrosion occurs when these highly reactive monatomic oxygen molecules erode aluminum and plastics.
Some materials commonly used in commercial interconnects are not suitable for spacecraft connectors. For example:
- 100% tin plating can grow “whiskers” that can cause catastrophic electrical short circuits.
- Silver plating is significantly affected by ATOX corrosion.
- Cadmium is unstable in vacuums.
- Plastics are very sensitive to ATOX as well as ionizing radiation.
The ideal protection against galvanic corrosion is employing the same material in both contacts: aluminum with aluminum, zinc with zinc, etc. Alternatively, it is helpful to employ materials with low galvanic potential, such as space-grade polymers, thermoplastic composites and glass (fiber optics). Plating and coatings can be used with alloys to avoid forming an electrolytic cell. Copper-alloy wiring and terminals exhibit relatively low galvanic potential when coupled with nickel, tin and silver. Gold (Au) plating resists ATOX corrosion because Au is a noble metal that normally resists oxidation. Silicon-dioxide coatings can protect polymers from ATOX corrosion because SiO2 is already fully oxidized.
Choosing the Right Connectors for Space
Since the 1960s, TE Connectivity has created space-grade connectors designed for high performance. TE solutions support LEO initiatives with space-qualified SpaceVPX, VITA, NASA, ESA and MIL-SPEC compliant products. And commercial-off-the-shelf (COTS) and COTS+ components help meet mission profiles on strict time and cost budgets.
Explore how TE’s innovative product portfolio meets the unique demands of low-Earth-orbit — from micro-miniature and nano-miniature connectors that overcome SWaP challenges to wire and cable that help control ESD.
Find Out How TE Can Support Your Next LEO Challenge